Jeff Jones, PhD
Postdoctoral Fellow
Herzog Lab, Washington University in St. Louis

Organisms have evolved endogenous circadian (~24 h) rhythms in behavior and physiology to anticipate reliable daily events such as the light cycle. These rhythms are synchronized to local time by the master circadian pacemaker, the suprachiasmatic nucleus (SCN). The SCN, with its well-defined inputs (light) and reliable outputs (daily rhythms) is a uniquely advantageous model in which to investigate the fundamental neuroscience question of how genes, neurons, and circuits interact to influence behavior and physiology. Moreover, dissecting the circuits that regulate circadian rhythms in a healthy brain is crucial to understand how the disruption of these rhythms contributes to disease. SCN neurons exhibit daily rhythms in firing rate and clock gene expression that, together, synchronize molecular and neuronal clocks elsewhere in the brain and body. Although the neuronal activity of the SCN is generally highest at midday, peak times of individual rhythmic outputs vary widely. Thus, my overarching
research goal is to understand how (an ostensibly uniform) circadian output from the SCN is encoded by downstream neurons to ultimately generate diverse endocrine, autonomic, and behavioral rhythms with different phases and waveforms. Does SCN firing induce or shift circadian gene expression and neuronal firing in subsets of target neurons? Do these neurons encode daily timing signals from different SCN neuron types? How do these neurons integrate circadian input with signals from other brain regions? And, ultimately, how do rhythms in these neurons regulate circadian outputs?
​
​
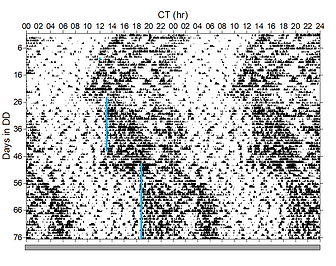
Fig. 1. Double-plotted actogram of a mouse expressing ChR2 in its SCN. Daily 1 h, 8 Hz stimulation (blue lines) is able to reset the circadian clock to entrain locomotor activity rhythms.
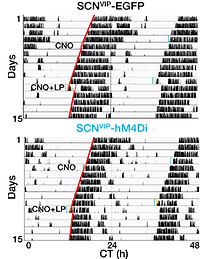
Fig. 2. Chemogenetic inhibition of SCN VIP neurons greatly attenuates light-induced phase shifts in locomotor activity.
My research to date has focused on understanding the inputs to, network regulation of, and outputs from the SCN that together generate circadian rhythms in behavior and physiology. The future of studying circadian circuits requires the use of techniques that allow for the manipulation and measurement of neuronal circuits. However, for these systems-level techniques to be useful for circadian neuroscience, they need to be implemented in specific cell types and circuits over the course of multiple days. To overcome this limitation, I have developed novel methods to non-invasively and continually measure in vivo rhythms in neuronal activity, gene expression, and hormone secretion while simultaneously manipulating firing rate or clock gene expression rhythms in individual animals over multiple days. These include the first applications in circadian biology of in vivo, cell-type specific, long-term (continually over 7+ days) imaging of neuronal activity and clock gene rhythms in the SCN and in SCN target neurons in combination with opto- and chemogenetic manipulation of SCN firing or CRISPR/Cas9-mediated disruption of clock genes. These methods are critical to understand how, mechanistically, SCN firing interacts with clock gene and firing rhythms in downstream neurons to regulate circadian physiological and behavioral outputs.
​
​
Research Directions
​
1. Defining the transfer functions for circadian output circuits.
​
2. Elucidating the neural basis of sex differences in circadian outputs.
​
3. Analyzing the robustness and sensitivity of the circadian system.
​
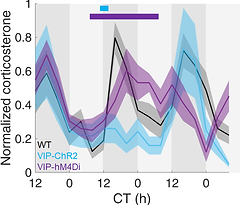
Fig. 3. Optogenetically activating or chemogenetically inhibiting SCN VIP neurons in vivo suppresses or enhances corticosterone rhythms measured continually over multiple days.
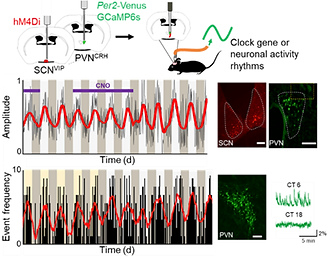
Fig. 4. Clock gene expression rhythms and calcium activity can be recorded continually over multiple days using in vivo fluorescent or bioluminescent fiber photometry.
As nearly every aspect of behavior and physiology is under circadian control, the long-term direction for my lab will be to apply these aims to endocrine (e.g., corticosterone), physiological (e.g., cardiovascular function) and behavioral (e.g., learning and memory) rhythms that each have their own phases and waveforms. Determining the coding strategies of these circuits will inform translational experiments investigating the circuit basis of circadian dysfunction that both leads to, and is a symptom of, disease.